Abiogenesis II: The Hen Or The Egg?
This article is the second in a series on the origins of life, abiogenesis, chemical evolution, and the RNA world hypothesis.
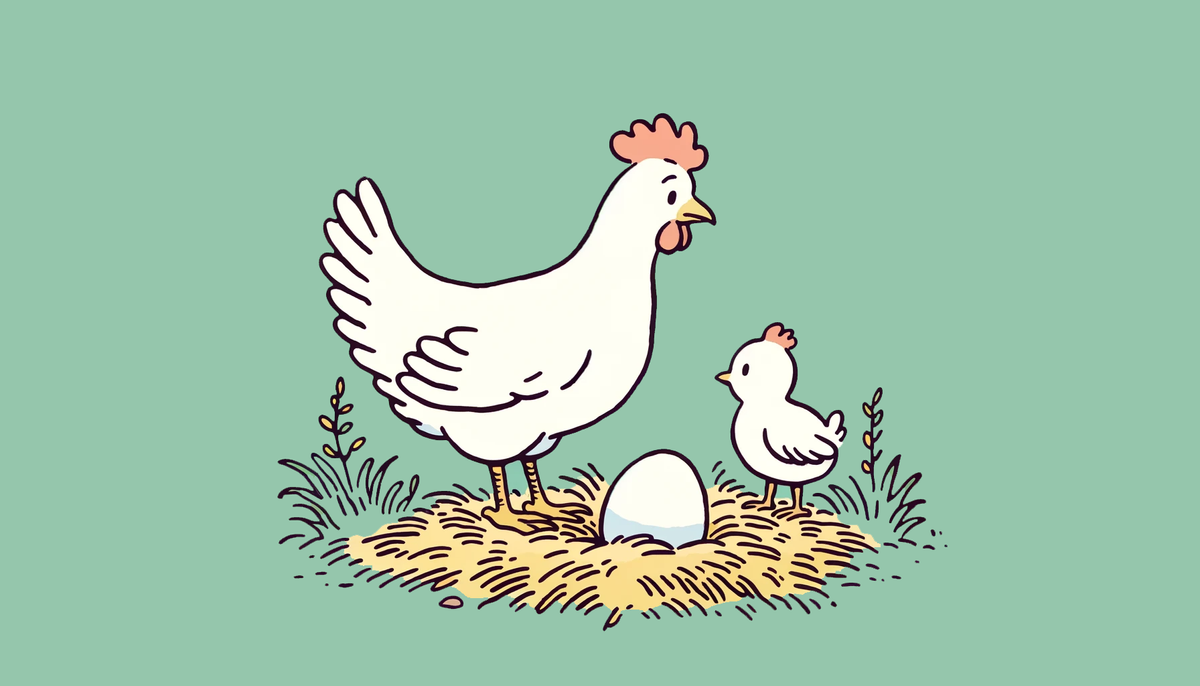
Now let us look at evolution from the other direction: In life today, there is a highly conserved code that we humans (partially) share with all living creatures, be they fruit flies or forest elephants. This code is not stored in RNA, but in the even more stable DNA, or deoxyribonucleic acid, in which ribose is replaced by the energetically more stable deoxyribose. Two molecules of DNA form a double strand, which also contributes to its stability: The nucleic bases cytosine and guanine, as well as thymine and adenine, have such a matching spatial structure that they attract each other electrostatically and hold the two strands together. However, one condition must be met for this to happen: Wherever there is cytosine on one strand, there must be guanine on the other strand, and vice versa. In the same way, where there is thymine, there must be adenine on the other strand, and vice versa. This means that the DNA of one strand within a double strand is always a copy of the other, with both running in opposite directions. If we abbreviate the nucleic bases by their initial letters, we can easily imagine such a possible double strand:
…AGCTAGCTAGCTAGCTAGCTAGCTAGCTAGCTAGCTAGCTAGCTAGCTAGCTAGCT…
…TCGATCGATCGATCGATCGATCGATCGATCGATCGATCGATCGATCGATCGATCGA…
A double strand of DNA then forms the well-known double helix structure, which is particularly stable, as described by James Watson and Francis Crick in 1953. Both were awarded the Nobel Prize in 1962.
In organisms known to us, the double strand of DNA can be separated for a short time by specific enzymes to generate a matching strand of RNA (it contains uracil instead of thymine, which matches also adenine). This new single strand of RNA - known as messenger RNA - can then be translated into a chain of amino acids (or a polypeptide chain) by a system in which three consecutive nucleic bases code for one amino acid. The memory code of DNA, which uses four different letters, thus becomes the functional alphabet of proteins with twenty letters (20 amino acids). The linear sequence of nucleic bases in DNA can thus be transcribed via RNA into an equally linear sequence of amino acids, true to dogma:

The polypeptide chain is the lowest level of organization of proteins. It forms three-dimensional structures: proteins. Because twenty different amino acids can be assembled as elements of an almost arbitrarily long chain, proteins can have a wide variety of chemical properties.
By the way, we call every unit of information in the DNA, whose transcription to the amino acid system results in a (meaningful) protein, a gene. Every manifestation of our body, from the course of neurons in our brain to the skin on our big toe, follows the expression of these genes and the specific interaction of the resulting proteins. Some proteins form the fibers of our tissue structures, such as collagen. Others are found in cell membranes, where they form channels, for example. Some even have pockets at key points in their backbones into which other molecules can bind, twisting the entire basic structure of the protein. For example, an ion channel may only open when a specific signaling molecule binds. Others are catalytic; we call them enzymes. Their basic functions need to be explained briefly: Enzymes provide microenvironments that can lower the activation energy of chemical reactions. This is possible because enzymes acquire special chemical properties: some bind to an inorganic molecule, such as a metal ion. This in turn can break chemical reactions into intermediate steps, each of which requires less energy. In turn, the metal ion forms short-lived complexes with the reactants. Thus, a reaction with high activation energy becomes several partial reactions with such low activation energy that the ambient energy is sufficient to initiate them. The metal ion is the ladder that makes it possible to climb a high wall. Enzymes can therefore catalyze chemical reactions - they are biocatalysts and as such are key to metabolic processes. They make it possible for energetically complex reactions to take place without lightning, volcanoes, or the like. Enzymes perform countless tasks in our organism and exist in thousands of different variations with different properties. Thousands of genes contain the blueprints for them.
The translation from DNA to RNA and from RNA to an amino acid chain (or protein) is done by special enzymes that catalyze all these chemical reactions. However, these translation enzymes are in turn produced by the expression of genes, which in turn is not possible without enzymes. How life could arise from the first primordial chemical compounds is the age-old hen-or-the-egg question. What was there first? The energetically stable chain of nucleic bases - the code for a protein - or a primordial protein capable of multiplying chains of nucleic bases?
Sidney Altman and Thomas R. Cech solved this puzzle when they made a groundbreaking discovery in 1982: They found a family of "enzymes", present in every cell, that did not consist of a polypeptide chain, i.e. amino acids, but of RNA, and called them ribozymes. Both were awarded the Nobel Prize in Chemistry in 1989 for their work.
Therefore, a complex RNA molecule can not only store information, but also potentially perform similar tasks as an enzyme. So maybe these ribozymes are living fossils from another world where RNA did all the work inside living organisms? According to this hypothesis, the question of the chicken (proteins) and the egg (DNA) would be solved: Neither came first.
After the emergence of nucleic bases and the first RNA molecules, chemical evolution probably moved in slow steps toward RNA-based life - an RNA world. By chance, longer and longer RNA molecules evolved, which in turn folded in space to form more complex shapes. However, the spatial structure of a long RNA molecule itself is not a product of chance, but follows rules derived from the primary structure of the strand, i.e., the sequence of the four nucleic bases. Think of DNA, where two matching nucleic bases attract each other to form a double strand. This rule also applies to a single strand of RNA: if an RNA is long enough, it is likely to have segments that match.
This also allows a single strand of RNA to attach to itself at different points according to the rules dictated by its nucleobase sequence, forming complex spatial structures. These sometimes take on functions similar to those of proteins. In the long breath of millions of years, longer and more complex RNA molecules evolved, and were probably the basis of all life.