Abiogenesis III: The RNA World
This article is the third in a series on the origins of life, abiogenesis, chemical evolution, and the RNA world hypothesis.
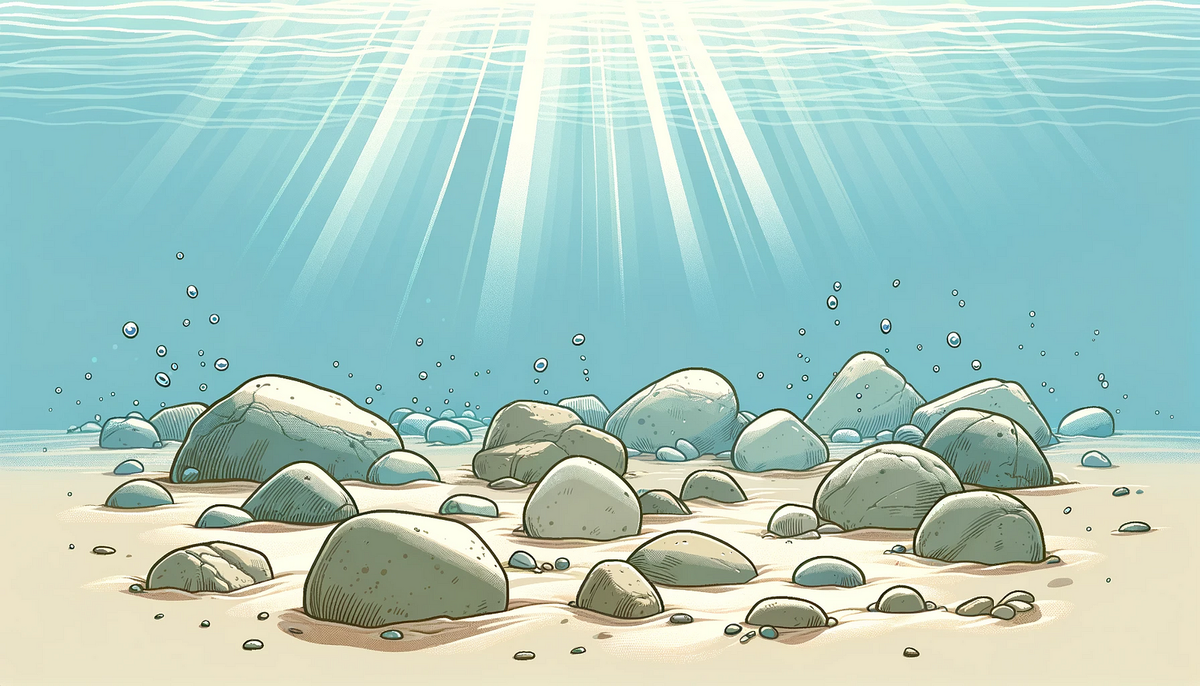
The exact definition of life has been debated in the scientific community for decades. In the following we will stick to the NASA definition:
“Life is a self-sustaining chemical system capable of Darwinian evolution”
Therefore, a chemical system must meet two preconditions: it must be able to organize itself and it must be able to reproduce itself. Many chemicals fulfill the first precondition. In a primitive way, even a blob of fat in meat broth is self-organizing: electrostatically uncharged (apolar) fat molecules form a sphere in water - the optimal surface-to-volume ratio - to reach the energetic optimum in the vicinity of electrostatically charged (polar) water molecules. Due to its lower density compared to water, a fat droplet will rise to the surface of the water. Upon reaching the surface, it transforms from a spherical shape into a thin circular layer, which is the most energetically favorable configuration - essentially forming a blob of fat.
Some fats, however, are only partially apolar: they are charged at one end and can interact electrostatically with water molecules (water-loving/hydrophilic), and uncharged at the other end (fat-loving/hydrophobic). We call them amphiphilic. They form a flat double layer in water, with the hydrophilic ends facing the water and the hydrophobic ends facing the center of the layer - a membrane!
To achieve the most energetically favorable state, the membrane encapsulates itself into a bubble, ensuring that none of the hydrophobic ends are exposed to water. These structures, known as lipid vesicles or liposomes, are essential to life. They separate an internal domain from the external environment, providing a critical basis for spatial organization and compartmentalization. Such amphiphiles, which formed the first liposomes, probably emerged abiotically in the vicinity of undersea volcanic eruptions, on mineral surfaces, and were able to create small confined spaces in the still dead primordial sea. At some point, the liposomes may have absorbed the RNA so that it was no longer directly exposed to the environment. Until now, an essential principle of chemistry has been ignored: entropy. By its very nature, every chemical system strives for maximum disorder. Maintaining order always requires energy to resist the constant gradient toward disorder. The more complex a system becomes, the greater the potential for disorder and the greater the energy required to maintain order. Liposomes made complex organization, and ultimately life, possible in the first place because they countered the three-dimensional problem of disorder with a two-dimensional boundary - a surface that limits space (we will come back to this).
We don't know the exact developmental steps of the abiotic origin of life, but let's explore a hypothetical scenario: Consider a liposome with all kinds of RNA buzzing around inside. This system is sealed off from the outside by a membrane, but it is still chaotic inside. Gradually, RNAs may form that have accidentally acquired catalytic properties: Ribozymes. It is plausible that the first ribozymes that appeared on the path to RNA-based life were capable of ligating (joining) two other RNA strands. We call such ribozymes (RNA) ligases. Even an RNA consisting of a chain of a few dozen nucleobases can theoretically act as a ligase. To understand this, we need to remember the properties of RNA: We recall that the base pairing of two strands of DNA also applies to RNAs (while two strands of RNA bind less stably). It follows that two different RNAs can form a double strand if they have matching nucleic base segments. Therefore, if an RNA ligase encounters two RNAs X and Y that match its own base sequence in essential regions, X and Y can "stick together" with the RNA ligase. If the ends of X and Y (almost) touch, a connection between the two strands can be catalyzed. Charged particles such as magnesium ions, which are sufficiently dissolved in the primordial soup and also in our liposome, enable this process by dividing the reaction into sub-steps, as mentioned above. Of course, our ligase cannot bind every combination of RNAs, only those that match its own structure. You can think of it this way:
Ligase
…AAGGCCUUAAGGCCUUAAGGCCUUGAGCUAGCUAGCUAGCUAGCUAGCU…
UUCCGGAAUUCCGGAAUUCCGGAA UCGAUCGAUCGAUCGAUCGAUCGA
X Y
UUCCGGAAUUCCGGAAUUCCGGAACUCGAUCGAUCGAUCGAUCGAUCGA
XY
Principle of an RNA ligase (oversimplified)
RNAs X and Y can now be ligated when cytidine triphosphate (CTP) is added, releasing pyrophosphate. Since the electrostatic bond that holds an RNA double strand together is weaker than that of a DNA double strand, the new RNA XY soon detaches from the ligase. The consequences of this principle are far-reaching: larger RNA molecules are formed that partially match the ligase that produced them. It seems possible that regular networks of ligases will eventually form in our liposome, reproducing each other.
Tracey L. Lincoln and Gerald F. Joyce published the results of an amazingly elegant experiment in Science in 2009: If, for example, one ligase A produces a second ligase A' from two smaller RNAs (Y' and X'), and this second ligase A' in turn ligates two other RNAs (Y and X) to an RNA that corresponds exactly to the first ligase A, then nothing less than a self-replicating system has been created. We are approaching the fulfillment of the two established conditions for life. However, since only individual molecules in our liposome can replicate, and not the entire system, it would be pointless to speak of life at this stage.
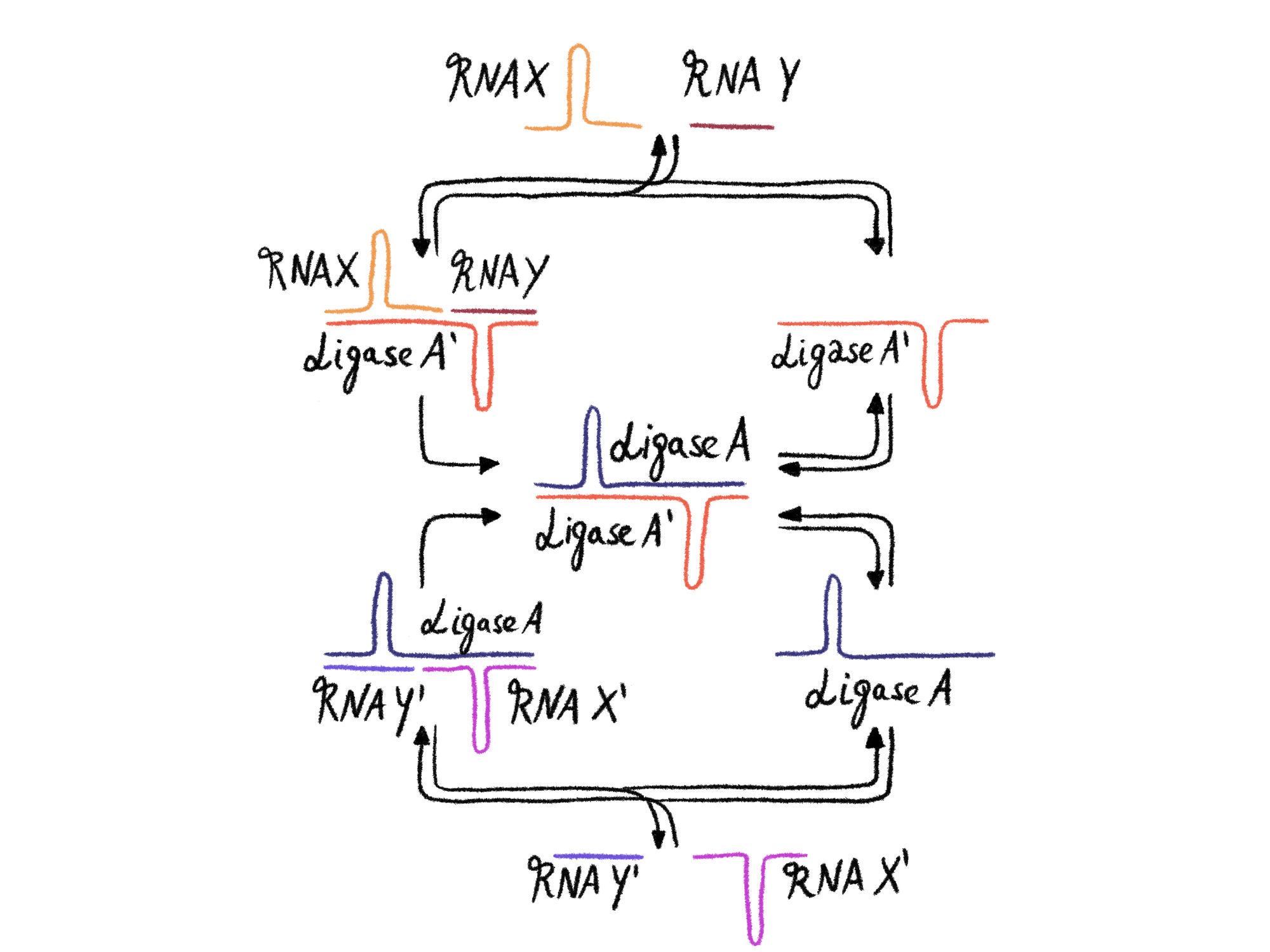
The evolution of the two-ligase system, while seemingly improbable, may actually be a natural progression from the emergence of the first ligase. The new RNA ligases are likely to share structural similarities with their parent ligase, predisposing them to function as ligases themselves. Consequently, it's quite possible that complex networks, such as the two-ligase system mentioned above, could evolve. To extend this idea, over long periods of time - and we've had plenty of time in the infancy of our planet - complex systems of ligases operating within liposomes could have evolved. Random processes could lead to the formation of larger ligases from smaller ones. Gradually, a sophisticated ensemble of interacting ribozymes could have evolved from the initial chaos of nonfunctional RNA.